Introduction:
Quantum computing represents a groundbreaking leap in computational technology, promising to revolutionize various fields by solving complex problems that are currently intractable for classical computers. Unlike traditional computers, which process information in binary bits (0s and 1s), quantum computers leverage the principles of quantum mechanics, using qubits that can exist simultaneously in multiple states. This capability allows quantum computers to perform many calculations at once, offering exponential speed-ups for certain tasks.
The concept of quantum computing was first proposed in the early 1980s by physicist Richard Feynman and computer scientist David Deutsch. Since then, the field has evolved from theoretical studies to tangible prototypes developed by leading tech companies such as IBM, Google, and Intel. Despite the impressive progress, practical quantum computing is still in its infancy, with significant technical and engineering challenges to overcome.
As the next frontier in technology, quantum computing holds the potential to transform industries including healthcare, finance, cryptography, and climate science. This introduction sets the stage for exploring how quantum computing works, its current state, potential applications, and the future landscape of this exciting technology.
How Quantum Computing Works
Quantum computing operates on principles fundamentally different from those of classical computing. At its core are qubits, the quantum counterpart to classical bits. While classical bits represent a binary state of 0 or 1, qubits exploit the phenomena of superposition and entanglement to exist in multiple states simultaneously. This enables quantum computers to process a vast amount of information in parallel, leading to potentially massive computational speed-ups for certain types of problems.
Superposition is a principle where a quantum system can exist in multiple states at once. For example, while a classical bit is either in state 0 or 1, a qubit can be in a state that is both 0 and 1 simultaneously. This allows quantum computers to perform multiple calculations at once, exponentially increasing their processing power as more qubits are added.
Entanglement is another critical quantum phenomenon where qubits become intertwined in such a way that the state of one qubit directly affects the state of another, no matter how far apart they are. This interdependence allows for incredibly complex and coordinated computational tasks, where the state of the entire system can be known by measuring just a few qubits.
Quantum computing also utilizes quantum gates, which manipulate the states of qubits through quantum operations. Unlike classical logic gates, which perform operations on binary bits, quantum gates perform operations on qubits using quantum mechanics principles. These operations form the basis of quantum algorithms, which are sets of instructions designed to solve specific problems by taking advantage of quantum mechanics’ unique properties.
One of the most famous quantum algorithms is Shor’s algorithm, which can factor large integers exponentially faster than the best-known classical algorithms. This has significant implications for cryptography, as many encryption systems rely on the difficulty of factoring large numbers. Another important algorithm is Grover’s algorithm, which provides a quadratic speed-up for unstructured search problems.
The differences between classical and quantum computing extend beyond just the hardware and algorithms. Quantum computers require extremely low temperatures to maintain the coherence of qubits, as any interaction with the environment can cause decoherence, leading to errors. This makes building and maintaining quantum computers a significant engineering challenge, but advances in this field are rapidly overcoming these obstacles.
Quantum computing represents a paradigm shift in how we process information, promising to tackle problems that are currently unsolvable with classical computers. As research and development continue to advance, the potential applications and transformative impact of quantum computing are becoming increasingly evident.
Current State of Quantum Computing
The current state of quantum computing is a blend of promising advancements and ongoing challenges. Over the past decade, major technology companies and research institutions have made significant strides in developing quantum computers and demonstrating their potential. Leading the charge are companies like IBM, Google, and Intel, each with their own approach and milestones.
IBM has been a pioneer in making quantum computing accessible. Their IBM Quantum Experience allows researchers and enthusiasts to experiment with quantum algorithms on real quantum processors via the cloud. IBM’s quantum computers, such as the IBM Q System One, have showcased capabilities with increasing qubit counts and improved coherence times. IBM’s Quantum Network also fosters collaboration, with over 100 organizations participating to advance the field.
Google made headlines in 2019 when it claimed to achieve quantum supremacy with its Sycamore processor. Quantum supremacy refers to the point at which a quantum computer can perform a calculation that is practically impossible for classical computers. Google’s Sycamore processor, with 54 qubits, performed a specific task in 200 seconds that would take the world’s fastest supercomputer thousands of years to complete. While this demonstration was specific to a particular problem, it highlighted the potential of quantum computing.
Intel is focusing on scalable quantum hardware. They have developed Tangle Lake, a 49-qubit superconducting quantum test chip, and are investing in various quantum technologies, including spin qubits and silicon-based quantum processors. Intel’s approach aims to leverage their expertise in semiconductor manufacturing to scale up quantum processors efficiently.
Despite these advancements, quantum computing still faces significant challenges. One of the primary issues is quantum decoherence, where qubits lose their quantum state due to interactions with their environment. This requires quantum systems to be maintained at extremely low temperatures and isolated from external disturbances, complicating the engineering and scalability.
Error correction is another critical challenge. Unlike classical computers, where errors are relatively easy to detect and correct, quantum error correction is complex due to the fragile nature of qubits. Researchers are developing sophisticated error-correcting codes and fault-tolerant quantum computing techniques to address this issue.
Additionally, software development for quantum computing is in its early stages. Creating algorithms that can fully utilize quantum hardware’s capabilities requires a deep understanding of quantum mechanics and computer science. Languages such as Qiskit, developed by IBM, and Google’s Cirq are emerging to help programmers develop and test quantum algorithms.
In summary, while quantum computing has made remarkable progress, it remains a nascent field with substantial hurdles to overcome. The collaborative efforts of academia, industry, and government are crucial in advancing quantum technology and realizing its full potential.
Applications of Quantum Computing
Quantum computing holds the promise of revolutionizing various industries by solving complex problems that are beyond the capabilities of classical computers. Its potential applications span multiple fields, each with the promise of transformative advancements.
Healthcare is one of the most promising areas for quantum computing. Quantum algorithms can significantly accelerate drug discovery by simulating molecular interactions with unprecedented accuracy. This capability allows researchers to identify promising compounds and optimize drug formulations faster than ever before. Additionally, quantum computing can aid in genomics by analyzing and comparing large genomic datasets, leading to personalized medicine and better understanding of genetic diseases.
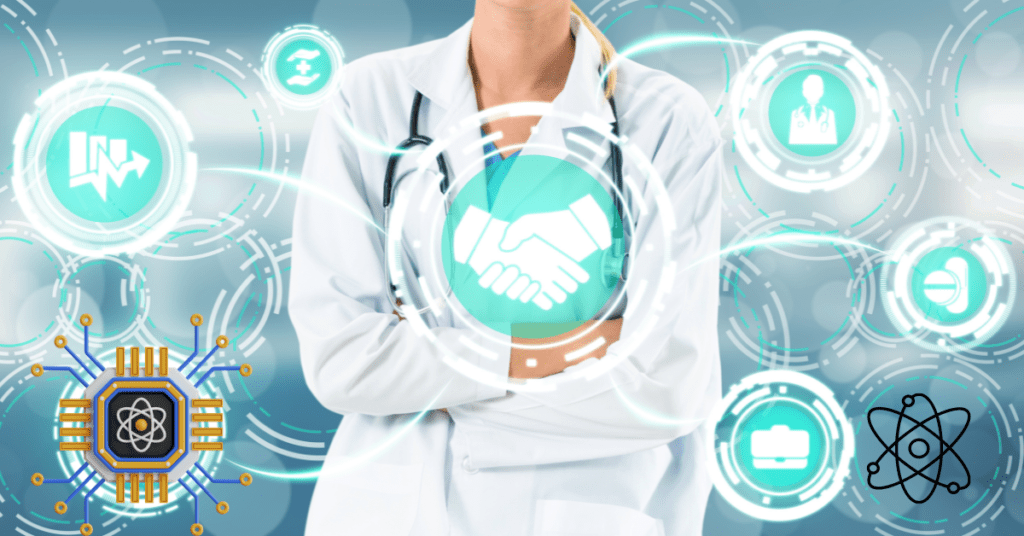
In the finance sector, quantum computing can enhance risk analysis and portfolio optimization. By processing vast amounts of financial data and performing complex calculations, quantum algorithms can provide more accurate risk assessments and identify optimal investment strategies. Quantum computers can also improve fraud detection by analyzing patterns in transactional data at speeds unattainable by classical systems, potentially preventing fraudulent activities before they occur.
Cryptography is another field poised for transformation. Quantum computing’s ability to solve complex mathematical problems, like factoring large integers, poses a threat to current encryption methods. However, it also offers opportunities to develop quantum-resistant cryptographic algorithms. Quantum key distribution (QKD) is an example of a quantum-safe encryption method that uses the principles of quantum mechanics to secure communication channels against eavesdropping.
Climate modeling and environmental science stand to benefit immensely from quantum computing. Accurate climate models require the simulation of numerous interacting variables, which quantum computers can handle more efficiently than classical supercomputers. This can lead to better predictions of climate change impacts and more effective strategies for mitigating environmental damage. Quantum computing can also optimize resource usage and energy distribution, promoting sustainability.
Artificial Intelligence (AI) and Machine Learning (ML) can be significantly enhanced by quantum computing. Quantum algorithms can process and analyze large datasets more efficiently, leading to faster and more accurate training of machine learning models. This can accelerate advancements in AI, enabling applications ranging from natural language processing to autonomous vehicles to become more sophisticated and reliable.
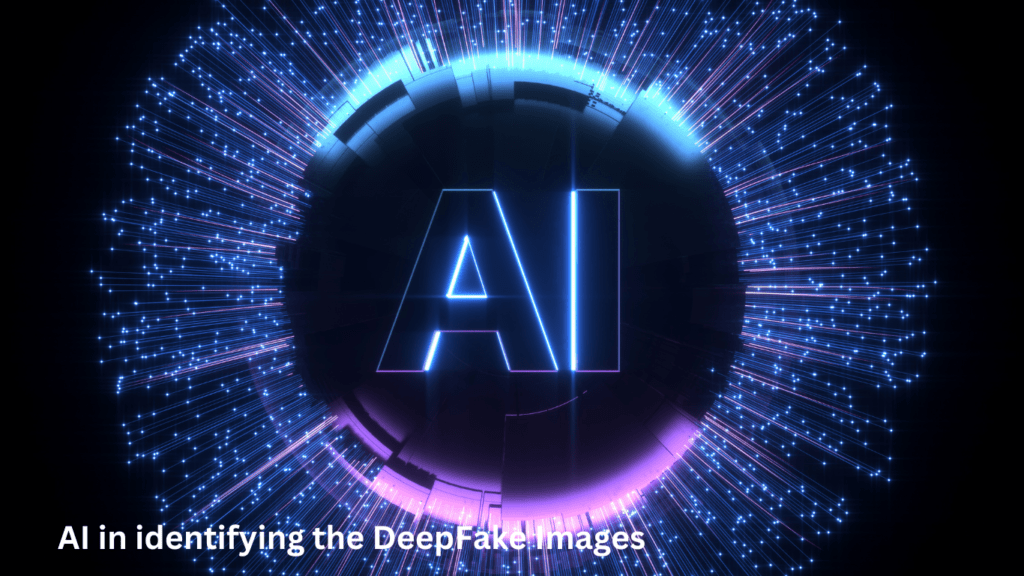
In the realm of material science, quantum computing can facilitate the discovery of new materials with tailored properties. By simulating atomic and molecular interactions at a quantum level, researchers can design materials with specific characteristics for use in electronics, energy storage, and more.
While the potential applications of quantum computing are vast, realizing these benefits requires overcoming current technical challenges and developing robust quantum systems. Nonetheless, the progress being made in quantum research and development indicates a future where quantum computing could fundamentally change how we approach and solve complex problems across various domains.
Future Prospects and Challenges
The future of quantum computing is both exciting and fraught with challenges. As research and development continue to progress, the potential for quantum computing to transform various industries becomes more apparent. However, several obstacles must be addressed before quantum computing can achieve widespread adoption and practical application.
Future Prospects:
One of the most promising aspects of quantum computing is its potential to solve problems that are currently intractable for classical computers. For instance, advancements in quantum algorithms could lead to breakthroughs in cryptography, enabling the development of unbreakable encryption methods. This would revolutionize cybersecurity, making data transmission and storage vastly more secure.
In drug discovery and healthcare, quantum computing could expedite the identification of new drugs and therapies by simulating complex molecular interactions. This would not only reduce the time and cost associated with bringing new medications to market but also open up new possibilities for personalized medicine based on individual genetic profiles.
The financial industry could also be transformed by quantum computing. Advanced quantum algorithms could optimize trading strategies, enhance risk management, and improve fraud detection systems. By processing large datasets more efficiently, quantum computers could provide deeper insights into market behaviors and trends.
In climate science and environmental modeling, quantum computing could offer more accurate simulations of climate patterns, leading to better predictions and strategies for combating climate change. This could result in more effective policies and technologies for environmental conservation and sustainability.
Challenges:
Despite these promising prospects, several challenges need to be overcome. One of the primary issues is the physical stability of qubits. Quantum computers are highly sensitive to their environment, and even minor disturbances can cause decoherence, leading to errors in calculations. Maintaining the necessary conditions for stable qubit operation, such as extremely low temperatures and isolation from external noise, is a significant engineering challenge.
Error correction is another critical challenge. Quantum error correction requires encoding information across multiple qubits, significantly increasing the number of qubits needed for reliable computation. Developing efficient error-correcting codes and fault-tolerant quantum architectures is essential for the practical deployment of quantum computers.
The scalability of quantum systems is also a major hurdle. Current quantum computers have relatively small numbers of qubits, and scaling up to thousands or millions of qubits will require new advancements in hardware and fabrication techniques. Achieving this scalability while maintaining qubit coherence and connectivity is a complex task that researchers are actively working on.
Software development for quantum computing is still in its infancy. Developing quantum algorithms that can leverage the full potential of quantum hardware requires a deep understanding of both quantum mechanics and computational theory. Tools and frameworks for quantum programming are being developed, but there is still a long way to go before quantum software development becomes as accessible as classical programming.
In conclusion, while the future of quantum computing is filled with immense potential, realizing this potential will require overcoming significant technical and engineering challenges. Continued collaboration between academia, industry, and government will be crucial in advancing quantum technology and unlocking its transformative capabilities.
Conclusion
Quantum computing is on the cusp of revolutionizing numerous fields by providing computational power far beyond the capabilities of classical computers. The journey from theoretical concepts to practical applications has seen significant advancements, yet the field remains in a nascent stage, filled with both promise and challenges.
The potential impact of quantum computing on industries such as healthcare, finance, cryptography, and climate science is immense. In healthcare, quantum computers can accelerate drug discovery and enable personalized medicine by simulating complex molecular interactions with unprecedented accuracy. The financial sector stands to benefit from enhanced risk analysis, optimized trading strategies, and more effective fraud detection. In cryptography, quantum computing poses a dual challenge and opportunity: while it threatens current encryption methods, it also enables the development of quantum-resistant cryptographic algorithms. Climate modeling and environmental science can leverage quantum computing to create more accurate models and strategies for combating climate change, leading to more effective policies and sustainable practices.
However, the path to realizing these benefits is fraught with challenges. Quantum computers are highly sensitive to environmental disturbances, making maintaining qubit coherence a significant engineering feat. Error correction remains a critical issue, requiring sophisticated techniques to ensure reliable computations. Scalability is another major hurdle, as current quantum systems are limited in the number of qubits they can effectively manage. Developing scalable quantum hardware and robust software tools is essential for the widespread adoption of quantum computing.
Despite these challenges, the collaborative efforts of researchers, technologists, and policymakers are paving the way for a future where quantum computing could fundamentally alter our approach to solving complex problems. The rapid advancements in this field suggest that, with continued investment and innovation, quantum computing will eventually transition from a specialized research area to a mainstream technology with transformative effects.
In conclusion, quantum computing represents the next frontier in technology and innovation, offering the potential to solve problems that are currently unsolvable. While significant challenges remain, the progress made thus far is encouraging. The ongoing efforts in research, development, and collaboration will be crucial in unlocking the full potential of quantum computing and ushering in a new era of technological advancement.
Related Content:
- How quantum computing works explained
- Applications of quantum computing in healthcare
- Future prospects of quantum computing technology
- Quantum computing challenges and solutions
- Impact of quantum computing on finance industry
Explore Micro2media
- The AI Revolution Continues: Introducing Meta AI
- Harnessing the Power of AI in Digital Marketing: Latest Trends and Innovations
- Revolutionizing Retail: AI-Driven Marketing Strategies for Unprecedented Success
- Wren: Building a Sustainable Future, One Action at a Time
- The AI Revolution in Business: A Deep Dive